The power of signalling
02 June 2020

How will Battery Health Reports support the remarketing of used Battery-Electric Vehicles (BEVs)? Experts from Autovista Group and TWAICE Technologies explain all in this whitepaper.
The BEV is a crucial building block in vehicle manufacturers’ mission to meet Europe’s CO2 targets. A strong supply of BEVs, which are currently heavily subsidised on the new-car market and which will mostly register in leasing agreements, will create a strong supply of used cars three to four years down the road.
Extended warranties for batteries merely represent a way to stop losses from a used-car buyer perspective: in the absence of certainty around replacement costs, these warranties help avoid a worst-case scenario where BEVs would become almost unsellable.
Next wave of BEVs approaches with force
The first two waves of battery electric vehicles (BEV) in 2011 and 2017 washed through Europe almost unnoticed. Market shares towards the end of 2019 were still well below 2%. The usual suspects for this underperformance are range, infrastructure, price and choice.
Market conditions are changing fast. Most recently launched BEVs demonstrate ranges above 400km and falling prices. Infrastructure is growing notably in the economically stronger countries; those will lead the transformation. OEMs have a serious stake this time in making electric mobility work; they are motivated by European fines of up to €35 billion, annually, for missing CO2 targets.
Hard to calculate without the host
Success on the new-car market is only possible if a model is successful on the used-car market. Why? A low price realised in the used-car transaction establishes a high loss in value that drives leasing rates up and in turn makes the car unattractive as a new car. However, high up-front incentives may stimulate demand for BEVs as a new car, particularly as in Germany, BEVs receive a €6,000 BEV bonus when purchased new and company car drivers can save up to 75% of a sizeable benefit-in-kind tax.
Nevertheless, the used-car buyers are the hosts of this party. Their willingness to pay a high price for the BEV as a used car will be low, if they fear that battery quality and range have deteriorated fast or will drop soon. Considerable information asymmetries exist between the buyer and seller of a BEV. OEMs have only partially addressed these concerns with extended battery warranties. By not providing transparency on the quality of the battery, they are missing an opportunity to develop the market and to create value.
The power of signals – bridging information asymmetry
Consider the quest for the ideal value for money in a hotel room. Guests are willing to pay a substantial premium for excellence in a hotel room if they have a chance to evaluate whether the offered price is adequate. They rely on evaluations from other guests. Powerful signals drive our buying decisions and willingness to pay for a product and services. We trust them when we look for the next mobile phone, when we shop for food or when we look for the right mechanic or when we seek medical advice. These signals come in the form of labels, certificates or ratings. External and independent parties endorse the most powerful ones. Some of them are mandatory; without them, the market would collapse. Some of them are voluntary, but no less powerful. When do signals work best?
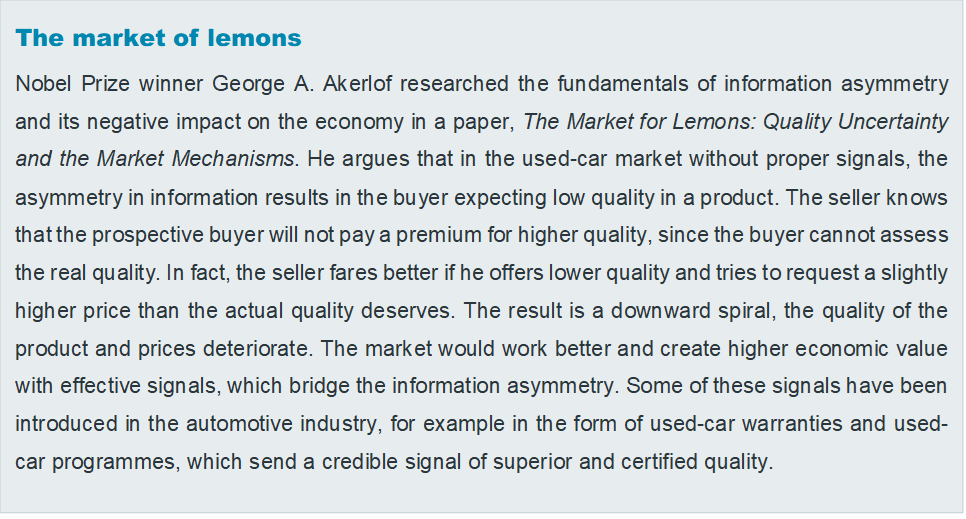
When they take out information asymmetry between buyer and seller. The financial impact of signals in the automotive industry is substantial. Autovista Group has evaluated the impact of extended warranties and used-car programmes on residual values. Five- and seven-year extended warranties drive the value of a used vehicle up by more than €500 for a 36-month old car in the volume segment.
Used-car programmes drive remarketing results up by approx. €400-600 per vehicle depending on segment and brand for the 36-months point in time. There are approx. 300,000 used vehicles sold every year under a used-car label in Germany within that age bracket. If each car delivers a €500 higher transaction price, this amounts to economic value for the German automotive industry of €150 million.
Batteries do not age gracefully – they like to be treated well
Battery treatment is the most important consideration in the assessment of used batteries, as it has a major impact on their degradation. Many of us are familiar with the cell-phone scenario. A new phone performs well initially, but after a while, depending on the model, this performance drops. The phone’s owner notices that they need to charge it more frequently, which has an impact on usage and will eventually mean they start thinking about buying a new phone. For many people, this will not hit their pocket too hard.
The same cannot be said for automotive batteries, as they can cost between €10,000 and 30,000 per pack – approximately 30-50% of the total vehicle cost. They are the most expensive singular component in a vehicle, whether it is new or used. As the battery operates and gets older, two major effects can be observed: firstly, the battery’s capacity gradually declines (limiting a vehicle’s range) and secondly, the inner resistance increases (causing a loss in performance).
A typical automotive lithium-ion battery, or battery pack, normally comprises serially connected modules, which are made up of cells. The cells consist of differing materials that need to interact with each other in a specific way for optimum battery performance. Over time, the impact of physical-chemical effects in the cells will affect the ageing process. Negative changes in the lithium-ion cells can occur on the anode, cathode, electrolyte, current collector, and the separator.
Not getting any younger
Lithium-ion battery electric vehicle (BEV) batteries are no exception to the rule. Huge amounts of time and effort are being invested into assessing the battery-ageing process. The goals of transparency for users and manufacturers and optimised service life drive this mission. Information on the battery’s state of health (SoH) is only available in very generic terms. The battery management system (BMS) will provide you with a number, such as 89%. First and foremost this tells you that 89% of the initial capacity is still usable. But is this a ‘good’ performance for a battery? This type of information alone is not a reliable indicator of battery ageing. This is like going to the doctor and being told that your current health is 89%.
What does that really mean for you? 11% of the missing ‘performance’ can translate into having a broken arm, or it could refer to a cardiac defect. Whilst the former will most likely not impact your overall life expectancy, the latter might be significantly reducing it. Without knowing any details about your habits, behaviours, and environment, the medic cannot make any specific prognosis about your life expectancy. More specific information is essential. Similarly, a reduced battery capacity can have different causes, and some will impact the remaining useful lifetime more than others. The state of health prognosis provided by the BMS falls far short of delivering the information required for decision making.
Vehicle and battery manufacturers alike have recognised the need to better understand the battery degradation process. Two kinds have been identified: cyclic (age resulting from battery use) and calendar (age resulting from chemical processes when the battery is not in use). Cyclic ageing is influenced by operating conditions (stress factors such as charging throughput, temperature, rate of power, charging/discharging hub and state of charge (SoC)) and the way in which the battery is charged. Calendar ageing is driven by the SoC and the storage temperature.
In the BEV, battery degradation manifests itself in two ways:
- The battery capacity starts to decrease, which reduces the range of an BEV
- Internal resistance within the cell increases, which weakens performance e.g. acceleration and charging.
Some of these degradation mechanisms can be influenced by the user, others cannot.
Manifestation of battery-ageing
One key physical-chemical effect involves changes to the anode/electrolyte interface. As soon as the anode comes into contact with the electrolyte solvent, a layer of film begins to form. This film is referred to as the Solid Electrolyte Interphase (SEI) and comprises the products of electrolyte decomposition. The SEI is still one of the least understood components of the battery cell. Although having an SEI is crucial for the battery’s safe operation it can have negative effects on battery-ageing. Essentially, the growth of this layer weakens lithium ions’ ability to react electrochemically. As the layer gets thicker, the mobility of lithium ions in the electrolyte reduces and the internal resistance increases. Further, lithium bound in the SEI cannot be used for storing energy any longer and thus leads to decreasing capacity.
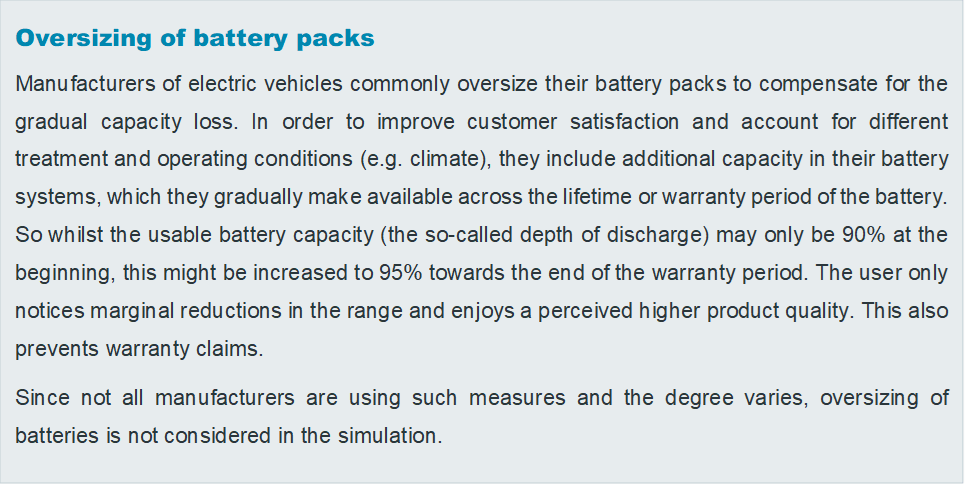
Lithium (Li-) platingis one of the most critical degradation mechanisms. Also known as lithium deposition, it concerns the build-up of metallic lithium around the anode of lithium-ion batteries mainly during (fast-) charging. This has two effects: an increase of the inner resistance and a loss of lithium inventory and thus capacity. It therefore adversely impacts both, performance and capacity. Ultimately, Li-plating can have a deadly effect on the battery. With an increasing amount of Li-plating, the capacity of a battery can rapidly decreases.
This so-called non-linear ageing occurs towards the end of a battery’s life before it becomes unusable. The decline is such that the battery cannot even be qualified as suitable for second life, i.e. the application in stationary storages for which lower currents and depth of discharges are required. This undesirable effect can be avoided by charging with moderate currents and avoiding very low temperatures. This is also the reason why some batteries with 80% state of health might already be a couple of cycles away from their end of life whilst others might still have hundreds of cycles to go. The potential buyer needs to consider the end-of-life factor – how many years does this battery have left? The range, i.e. the capacity, of the battery is not the only indicator.
The feel-good environment for batteries
The cell temperature is one of the most important influences on battery degradation. A lithium-ion battery’s feel-good area is very much like a human’s and lies between 20°C and 40°C for most of those commercially available. A temperature increase of about 10°C leads to chemical processes running twice as fast as before. If the temperature rises above 40°C, the rate of ageing increases incrementally, leading to a significantly reduced expected lifetime.
The opposite situation can be even worse news for batteries. Charging or discharging lithium-ion battery packs at cold temperatures may cause Li-plating and effect severe capacity loss. Ambient temperature is not the only culprit; battery cells themselves warm up during charging and discharging and must be effectively tempered.
In this paper, we do not consider the impact of ambient temperature on batteries. The stage in our case is set in Germany in a moderate climate. More extreme conditions – e.g. tropical or arctic – also have a significant impact.
Manufacturers address the temperature impact with the appropriate thermal management systems. The quality of the thermal management varies, and the correct setup is very complex, but generally the BEV user has minimal influence on this.
The use and misuse of batteries
This is different when it comes to the use of the vehicle. When using a BEV, the two degradation modes should be kept in mind: cyclic and calendar ageing. The former is significantly impacted by the user behaviour.
Obviously, a higher energy consumption due to speeding, rapid acceleration or mechanical braking instead of recuperation will ultimately cause the range of a BEV to reduce – like it does in a regular petrol car. Batteries must then be recharged more frequently, which drives up the overall cycle count. This means that the degradation occurs more quickly. Depending on the operating conditions, e.g. how much capacity of the batteries is used – the depth of discharge – or the ambient temperature, the cycles can also differ in their impact on the remaining useful lifetime of the battery.
As fast charging is often the biggest stress factor on a BEV nowadays, it is worth examining this element as well. BEV drivers who cover long distances and are under time pressure – think sales rep racing up and down the country to visit clients – may need to use fast charging regularly.
On the other hand, a BEV driver who takes their car out once or twice a week to visit their relatives down the road can easily use regular charging at home without putting any excess stress on the battery.
Again, the depth of discharge plays a role here. But it is also relevant to look at the target state of charge. Many manufacturers will, by default, only charge the vehicle to 80%. The reason is that the extremes – either completely discharged or completely charged – stress the battery significantly more than use in the middle of charge window (e.g. 30-60%).
Another effect comes into play here too – the calendar ageing. Parking a BEV fully charged is not ideal. When storing a battery or parking a BEV, lower states of charge and temperatures are better to prevent this degradation mode.
Battery-ageing simulation – comparing three treatments
As established, batteries are sensitive, and multiple factors (temperature, mileage, geography, topography) influence their ageing process. For the purposes of this paper, however, we focused on three specific factors – driving, charging, and parking behaviour.
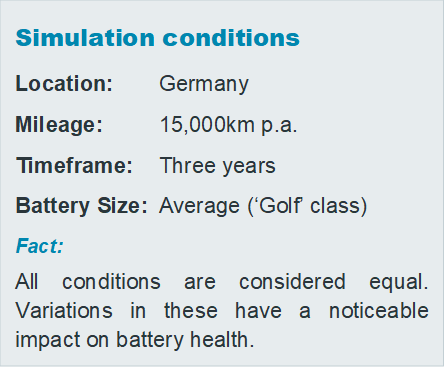
Why? Because we know that the usage behaviour of the BEV driver – how they drive, to what intensity level they charge their battery, how much electricity is consumed during driving time (e.g. impact of use of air conditioning) – is one key determinant of battery degradation.
TWAICE battery experts designed and carried out a simulation with the aim of better assessing the impact of battery treatment and its financial impact. Three levels of treatment behaviour were investigated:worse, average and better battery treatment. All relate to and have a (greater or lesser) effect on the degradation of a BEV battery.
Table 1: Three simulated treatments (all other factors constant)
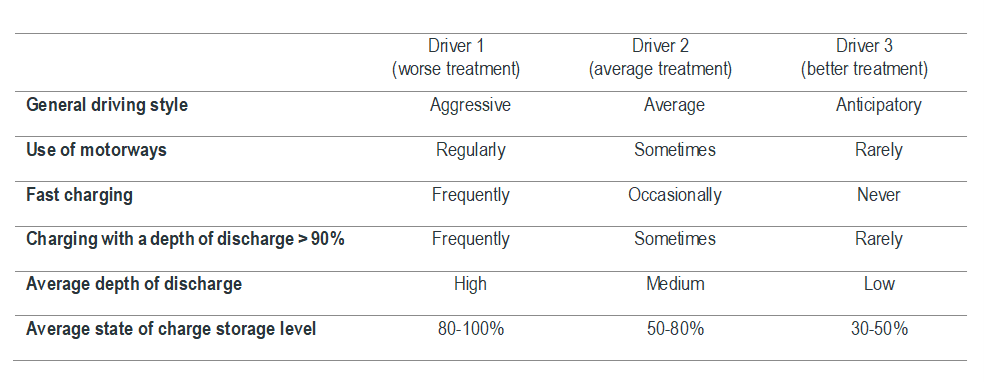
For the purposes of the simulation, the border conditions – temperature, mileage, geography – were all constant. We assumed an annual mileage of 15,000km over three years. The simulation represents driving in Germany (no speed limits on large parts of the national motorway system, temperate northern European climate).
Driver 1 represents worse battery treatment. Their general driving style can be termed aggressive; this kind of driver might be inclined to accelerate sharply when the light turns green, for example, and rarely practices regenerative braking. Factors such as traffic conditions and time of day will influence the degree of ‘aggressiveness’ – heavy traffic, for example, will automatically reduce it.
Probably under time pressure and with a lot of mileage to cover, they use the motorways a lot, which means frequently fast charging their BEV. The average depth of discharge is correspondingly high. These drivers may well suffer from ‘range anxiety’, which will impact their charging treatment – the main motivation being never to run out of range. The state of charge storage level is between 80% and 100%.
Driver 2 is our Joe Average, representing average battery-treatment behaviour and an ensuing neutral effect on battery-ageing. These drivers are in the grey zone between their aggressive and gentle counterparts. In a real-world scenario, they might be conscious of the recommended conditions and behaviour for BEV drivers and will generally try to adhere to these; however, unplanned events sometimes make this impossible. They are mixing the urban trips with some long-haul motorway journeys.
Driver 3 is at the other end of the scale and displays better battery treatment behaviour. This kind of driver is likely to take full advantage of the potential to regenerate energy, a concept known as eco-driving. Their anticipatory driving style is gentle, which can be attributed to their lifestyle and the factors affecting it: compared to Driver 1, they may rarely feel under time pressure and never carry out fast charging, presumably, because they never need to.
Figure 1: Battery state of health (SoH) over time
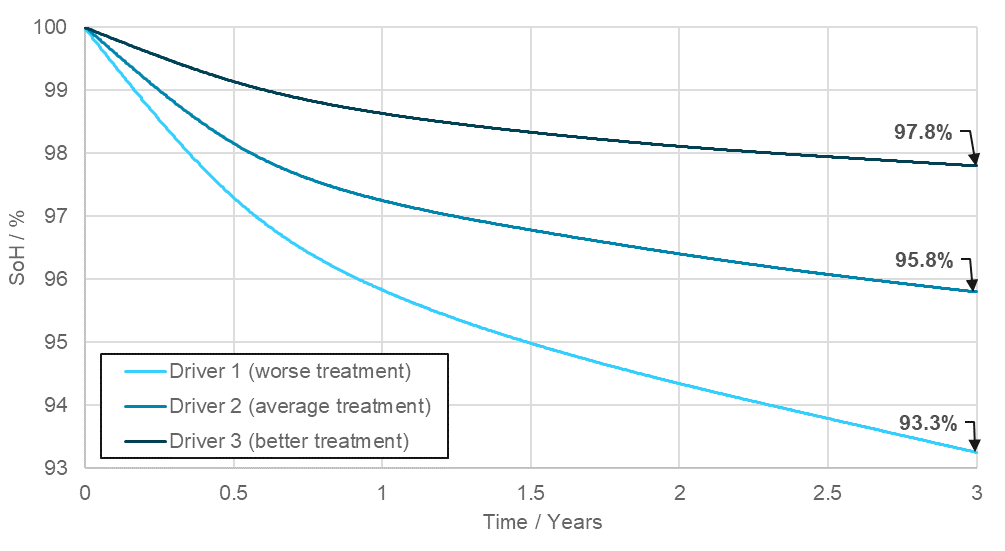
Figure 1 shows the SoH development of an average-sized battery under the three simulated treatment conditions (worse, average, better battery treatment). While all three SoH show a gradual linear decrease after year one, the graph clearly depicts how high-intensity battery treatment can make a considerable difference – as much as 4.5%-points – to battery capacity at a 36 months point in time. The simulation also enables predictions of what happens thereafter, we see even bigger differences. Assuming the state of health of 80% to represent the end of life, we see a spread of 41% end-of-life difference between the worse and the better treatments (see Table 2).
All the above demonstrates how beneficial it would be to assessors of BEV residual values to have access to (1) the treatment history of a battery and (2) prediction information regarding remaining battery life. Gaining data on the operating condition of a battery is key to assessing the remaining useful lifetime – this much is clear. But how can this information be obtained? This section addresses the question and provides a possible solution.
Table 2: End-of-life predictions
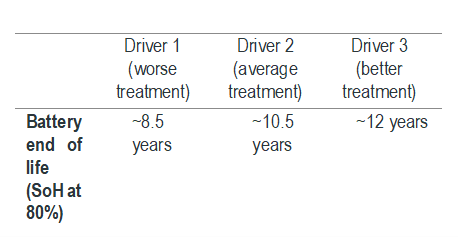
Data is the essential enabler of understanding battery condition and the remaining useful lifetime.
As established, capturing battery data is essential to providing information on the current condition and the remaining useful lifetime. But what kind of data are we actually talking about?
Battery status: Capacity
A first indication on the quality of the battery can already be provided by driving a so-called full cycle with the battery, i.e. completely charging and discharging it, to identify its capacity and the corresponding range. The internal SoH might also be an indicator but can also be misleading. The information on the current capacity does provide the information about the available performance at that time but cannot provide any information about the remaining useful lifetime.
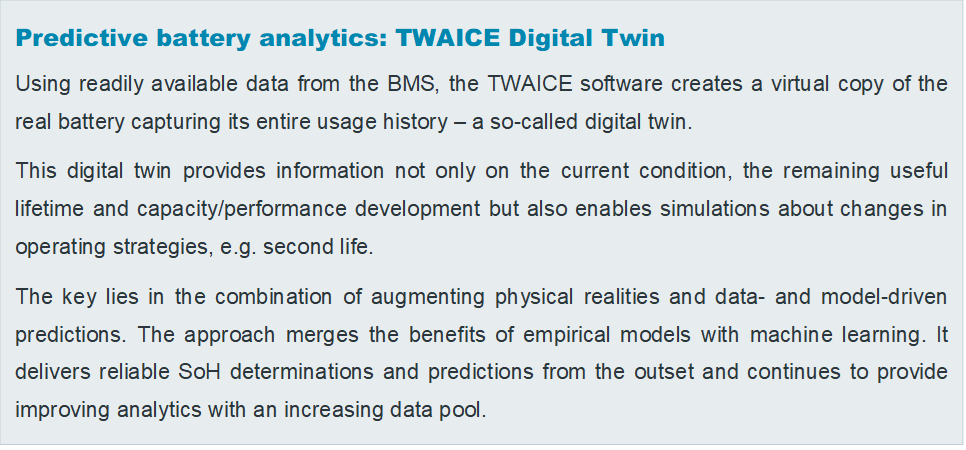
Battery history: Load characteristics and operating conditions
To enable the assessment of the used battery and its remaining useful lifetime, the consideration of its use is essential. This information ranges from the total energy throughput and cycle amount to the depth of discharge, the energy throughput at each time (e.g. charging), to the temperatures within the system. Whilst the data is always generated by sensors in the battery system and used by the BMS to prevent malfunctions, the information is not always saved or made available. In addition to the battery-system data itself, the operating conditions of the BEV also play a role. Climate, to just name one, can have an adverse effect on a battery exposed to excessive heat, humidity or cold. Such factors (except for actual temperature changes) are not captured by regular systems but their effect can be identified by assessing the electric-thermal behaviour of batteries.
In summary, the availability of detailed data from the vehicle and the battery can make a big difference in the assessment of used vehicles. From rough indications on the quality (e.g. current capacity) to precise predictions about the remaining useful lifetime and capacity as well as performance developments, the assessments depend completely on data.
Battery impact on used BEV evaluation
Currently, without signals or certificates for the actual battery quality (SoH), most recently launched C-segment BEV models will be trading at approx. €18,450 on average after three years, representing 47% of the average original list price.
Figure 2: Average C-segment BEV forecast RV, 36months / 45,000km, trade, Germany; latest generations Hyundai e-Ioniq, Nissan Leaf, Volkswagen e-Golf
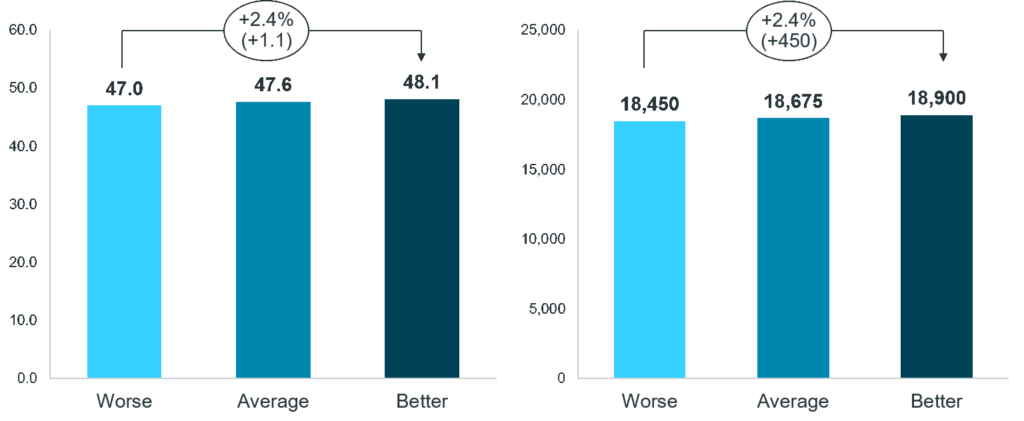
Providing a Battery Health Certificate (BHC) with a proven battery quality of 98% SoH, with a strong signal of a battery’s remaining capacity, could significantly increase RVs for BEVs. A higher SoH directly correlates with the possible range of the electric car. The 5% higher SoH of a better-treated battery represents not only a 5% greater range but also lower future degeneration of the battery and thus the range, which will be appreciated by the future owner. Autovista Group estimates that 1% SoH improvement (achieved via the stated driving profiles) equals an RV improvement of c. 0.5%. RVs of three-year old latest-generation C-segment BEVs would achieve a 2.4% uplift for better-treated batteries between the worst and the best treatment scenario. This represents a €450 increase in price for a typical vehicle (Figure 2). The financial impact of a higher-signalled battery quality is substantial. In the C-segment, a 2.4% higher used-car price results in a €4.5 million improvement for every 10,000 used BEV transactions.
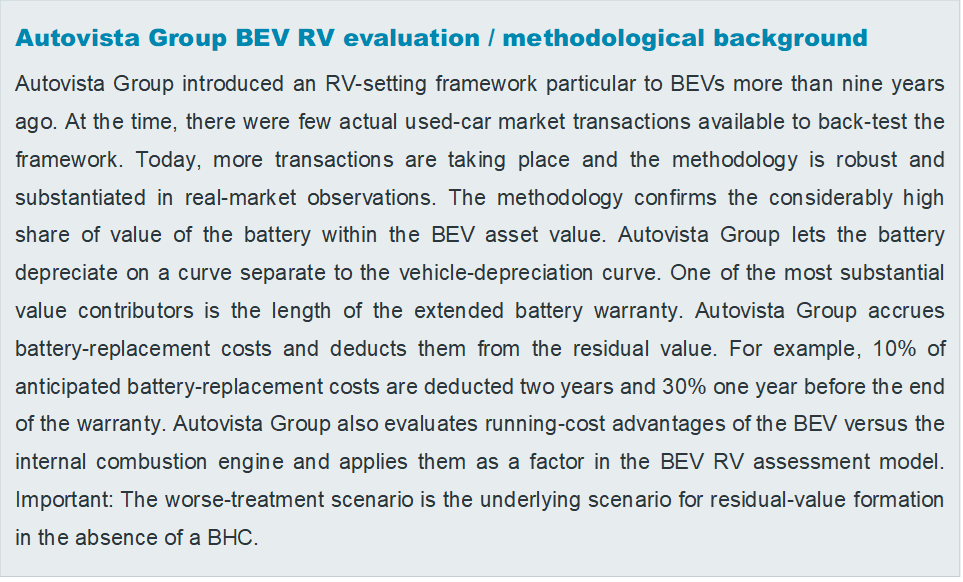
What should you do?
Currently, without a signal that indicates the battery quality, used-car buyers assume BEV batteries are worsely treated by the previous owner that in fact they are and, therefore, are only willing to pay a comparatively low price.
The Battery Health Certificate (BHC) could overcome these assumptions of worse-treated batteries, prove higher battery qualities and thus higher ranges.
- Used-car buyers should insist on obtaining a BHC to establish proven, independently assessed battery quality and corresponding range for the electric vehicle to be bought.
- The BHC should not only provide the actual SoH of the battery but also its treatment history, how often it was fast charged, how often it was highly discharged, the average depth of discharge, the average state of charge storage levels, as well as the expected remaining useful lifetime. These figures should all be compared to average battery treatment.
- Used-car sellers should provide a BHC alongside the description of the vehicle to achieve the maximum resale price, especially for a BEV with a better-treated battery, to prove the high quality of the battery and the still considerable range of the BEV to be sold.
- OEMs should be more transparent about battery health and enable analyses – this would add up to 2.4% in RV performance (for better battery treatment).
- Fleet and leasing companies could incentivise better treatment of batteries together with fleet managers and grant a kickback, similar to that for credit mileages. Worse treatment could be charged for, as with excess mileage.
- With a BHC in place for all used BEVs, residual values would rise as the worst-case assumption on battery treatment is moderated and eventually lifted by the BHC.
If you want to download a PDF of this article, click here. If you want to find out more about TWAICE, Autovista Group and how we can support your quest to improved BEV remarketing, visit our websites or contact the authors of this whitepaper.
Authors
Autovista Group
- Lead author – Ralf Sulzbach, Senior Project Manager Consulting, Autovista Group
- Contributors – Dr. Christof Engelskirchen, Chief Economist, Autovista Group
- Special thanks – Dr. Sarah Walkley, Chief Research Officer, and Karen Beynon, Managing Editor, Autovista24
TWAICE
- Lead author – Lennart Hinrichs, Commercial Director, TWAICE
- Contributors – Jonas Keil, Battery Engineer, TWAICE, Anna Lossmann, Marketing Manager, TWAICE, and Tim Neumeier, Battery Engineer, TWAICE